Photochemistry of Organic Aerosols
Chemical processes that convert volatile organic compounds (VOC) into secondary organic aerosols (SOA) are generally quite fast. For example, terpenes rarely last longer than an hour after they are released by vegetation on a hot sunny day. Once the condensable products of VOC oxidation partition into aerosols, the chemistry slows down considerably. However, it is incorrect to view SOA as a static collection of organic molecules which undergoes no chemistry after the initial particle condensation. A number of interesting “aging” processes leading to changes in the chemical composition and physical properties of aerosols occur on time scales ranging from minutes to days. Our group investigates mechanisms of chemical and photochemical aging in secondary organic aerosols using a combination of spectroscopic and mass spectrometric techniques.
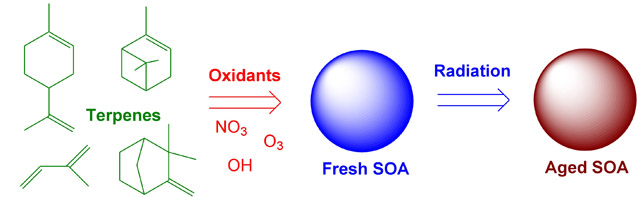
Direct photochemical aging refers to processes initiated by absorption of solar radiation within the particle. This aging mechanism can be effective only if the following conditions are satisfied: (1) the organic aerosol material must have significant absorption in the tropospheric actinic window; (2) the yields for condensed-phase photochemical reactions, such as photodissociation, must be large compared to that for fluorescence, vibrational relaxation, geminate recombination, and various other non-reactive processes. We have found that SOA formed by ozonolysis and photooxidation of VOCs do indeed absorb light at atmospherically relevant wavelengths [107], leading to rich photochemistry within the particles such as direct photodissociation of peroxide [50] and carbonyl [57, 60] functional groups.
We have demonstrated the importance of condensed phase photochemistry in aging of organic aerosols. For example, we found that condensed-phase photochemical processes in alpha-pinene SOA result in a decrease in mass and diameter of particles occurring on atmospherically-relevant time scales [98]. Photodegradation of SOA produces a variety of small, oxygenated VOCs, which evaporate from the particles leading to the observed mass loss. This photodegradation process could produce upwards of a few Tg/year of formic acid in the atmosphere – comparable to its primary sources [111]. However, uptake of VOCs during irradiation is also possible in a so-called photosensitized process. We recently showed that photosensitized uptake of limonene occurs in competition with the photodegradation, but that the photodegradation occurs on a much faster time scale, and is therefore more important aerosol aging [122].
Another avenue of our research is photochemistry of SOA in aqueous environments, which is relevant for understanding cloud-processing of organic aerosols. In our first paper on this topic, we reported the effect of UV irradiation on the molecular composition of aqueous extracts of limonene ozonolysis SOA. We showed that photolysis had a significant effect on the composition of the dissolved organics: oligomeric compounds were destroyed, carbonyl compounds were photolyzed, carboxylic acids were generated during photolysis, and large organic peroxides were recycled into smaller peroxides. In a related study on high-NOx isoprene SOA [80] we discovered new photochemical reactions converting organic nitrates into unusual heterocyclic compounds containing nitrogen. In our follow up studies, we showed that aqueous photodegradation is common to a broad range of SOA [93, 100, 118]. We found that the SOA material became more volatile on average after the photolysis [118]. These results suggest that SOA dissolved in cloud/fog droplets should undergo significant photolytic processing on a time scale of hours to days, and may need to be considered by atmospheric models.
Our group also performs experiments on photochemistry of SOA material in a variety of matrices, where we control the viscosity of SOA (by adjusting temperature and humidity), and/or the embedded molecules. To investigate the role of the particle viscosity on photochemistry, we investigated photolysis of various molecules embedded in alpha-pinene SOA at high (liquid) and low (glassy) temperatures and showed that photochemistry is suppressed in the glassy state [91]. In a follow up study, we studied this effect in three types of SOA and found that the photochemistry is suppressed at lower temperature or lower relative humidity in all three model systems [110].
We also conduct fundamental studies of photodissociation of important atmospheric molecules in water and ice. For example, we found that photolysis of the simplest organic peroxide CH3OOH embedded in ice clusters results in rich photochemistry on a picosecond time scale [63]. This was followed by a combined experimental and theoretical investigation of photodissociation quantum yields and absorption cross sections of CH3OOH in water and in ice [78]. Another study involved the absorption spectra and aqueous photochemistry of alkyl nitrates. We determined that the aqueous photolysis of the alkyl nitrates was insignificant compared to the gas-phase photodegradation and aqueous OH reactions [104]. Finally, we have investigated the mechanisms of photochemistry of cyclohexanone, an atmospherically-relevant carbonyl compound [115].
As opposed to SOA, which appear in the atmosphere as a result of VOC chemistry, primary organic aerosols (POA) are released directly into the atmosphere by their sources. POA include sea-salt aerosol particles generated by wave-breaking, soot particles produced by internal combustion engines, smoke particles produced by biomass burning, dust particles produced by re-suspension, and smelly particles emitted in the atmosphere by the cooking industry. Such particles are often decorated by an outer layer of fairly hydrophobic organic material such as phospholipids, fatty acids, and aromatics. This layer is slowly oxidized by OH, ozone, and nitrogen oxides in a process known as "chemical aging". We are interested in understanding the role of direct photochemical processes in processing fresh and aged POA particles. We have previously studied photodegradation of partially oxidized unsaturated fatty acids [47] and self-assembled monolayers (SAM) [43,46] as a model of photochemical aging occurring in POA particles. We discovered that reactions taking place during the oxidation of unsaturated films and SAM transform them into a photochemically active state capable of photolysis in the lower atmosphere. We think that photochemistry occurring in the oxidized aerosol contributes significantly to their aging. Our current efforts in this area focus on investigation of surface-specific photochemistry in SAM containing carbonyl and peroxide functional groups.
|